A GP guide to newborn bloodspot screening
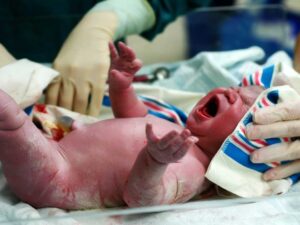
Need to know:
- The Australian newborn bloodspot screening (NBS) programs cover at least 32 conditions including congenital hypothyroidism, cystic fibrosis, congenital adrenal hyperplasia, spinal muscular atrophy, severe combined immunodeficiency and a range of inborn errors of metabolism.
- Not all countries offer newborn bloodspot screening programs. It is worth considering that infants who are new arrivals to Australia may not have been screened.
- The prenatal period is an appropriate time to educate parents about NBS, so they are informed and prepared for when testing is offered to their infant.
- It is important to verify at subsequent checkups that families have been offered NBS for their newborn, and that this has been completed for those who have consented.
Newborn bloodspot screening is a funded program offered to all Australian babies that aims to save brains and lives. Which conditions will be included in the screening has traditionally been decided based on the criteria developed by Wilson and Junger.1
Currently, the Australian newborn bloodspot screening (NBS) program screens over 99% of newborns annually, totalling over 300,000 infants each year.2 Among these, approximately one in every 1000 receives a diagnosis via NBS.3
This initiative traces back to the 1960s, when Dr Robert Guthrie utilised heel prick blood sample collection on filter paper to detect phenylketonuria.4 This gave rise to the colloquial name ‘Guthrie cards’ still used by some to describe the NBS test.
The NBS program has expanded beyond phenylketonuria, with tests now available to detect a number of inborn errors of metabolism, including congenital hypothyroidism, which is the most common condition identified.
This article focuses on the current landscape of NBS in Australia, the latest developments in conditions for which screening is available, and provides an overview of the screening process. It will review the crucial role of GPs in enhancing the program’s effectiveness, the challenges that persist and explore future possibilities in this evolving field.
Current landscape
Since its inception, there have been significant advances in Australia’s NBS initiative. The introduction of tandem mass spectrometry in the late 1990s revolutionised the process, enabling simultaneous screening for multiple conditions from a single blood sample.5 As a result, NBS programs in Australia have identified thousands of children with early, treatable conditions, preventing intellectual disability, morbidity, and in some cases, death.
While the specific conditions screened for vary slightly between regions, the program currently covers at least 29 conditions, including congenital hypothyroidism, cystic fibrosis, amino acid disorders, organic acid disorders, and fatty acid metabolism disorders.3
Other countries have also expanded their NBS panels. While the way conditions are counted between programs is not uniform, it is useful to look at other programs for benchmarking against the Australian programs.6
Some jurisdictions in the US screen for up to 35 different disorders, EU countries screen for between seven and 40 disorders, while Canada screens for between 14 and 36.7-9 The UK maintains a more conservative core NBS panel, screening for nine disorders.9,10
Sadly, NBS does not have global coverage. This is worth considering for infants arriving in Australia who may not have been screened in their country of birth.
In Australia, each state and territory government funds and operates its own NBS program. Five major laboratories located in NSW, Queensland, SA, Victoria and WA conduct the testing of dried bloodspots.
For regions without their own screening laboratories, samples are sent interstate for analysis.3 Since the 1980s, the five screening laboratories have collaborated through a joint committee established by the Royal Australasian College of Physicians and the Human Genetics Society of Australasia.11
Expansion of Australian newborn bloodspot screening programs
The Australian Government has made significant investments to expand the NBS. The primary objectives are to increase the number of conditions screened and ensure national consistency and equitable access for all newborns.12 All NBS programs have recently expanded to include screening for congenital adrenal hyperplasia, spinal muscular atrophy, and severe combined immunodeficiency.13
A new decision-making pathway has been established to streamline the process of adding new conditions that promotes transparency, consistency, and collaboration that includes opportunities for public input and consultation with various stakeholders, including families, clinicians, and advocacy groups.12
With the shift in public attitudes towards recognising the individual benefits of NBS beyond its public health goals, many families now understand that early diagnosis of inherited conditions, such as cystic fibrosis, can inform future reproductive decisions.
This shift in redefining the value of NBS programs has led to the inclusion of rare disorders, even when the potential impact on quality of life or life expectancy is limited.14 These attitudes will likely continue to evolve as the new era of genomic NBS emerges in the next decade.
Table 1 shows the 32 target conditions screened in Australia’s NBS programs at the time of writing. It is worth noting the additional conditions are under review for potential implementation, so check local resources for their relevant inclusions.
Table 1. Target conditions currently screened for by all jurisdictions in Australia’s newborn bloodspot screening programs
Condition type | Condition name |
Endocrine | • Congenital adrenal hyperplasia (21-hydroxylase deficiency) • Primary congenital hypothyroidism |
Metabolic | Amino acid disorders • Argininosuccinic aciduria • Citrullinaemia type I • Homocystinuria • Maple syrup urine disease • (Classic) phenylketonuria — including hyperphenylalaninaemia (phenylalanine hydroxylase and pterin enzyme deficiencies) • Tyrosinaemia types II and III • Remethylation defects Fatty oxidation disorders • Carnitine acylcarnitine translocase deficiency • Carnitine palmitoyltransferase I deficiency • Carnitine palmitoyltransferase II deficiency • Carnitine uptake defect • Long-chain 3-hydroxyacyl-CoA dehydrogenase deficiency • Medium-chain acyl-CoA dehydrogenase deficiency • Trifunctional protein deficiency • Very long-chain acyl-CoA dehydrogenase deficiency Organic acid disorders • 3-hydroxy-3-methylglutaric aciduria • β-ketothiolase deficiency • Glutaric acidaemia type II (multiple acyl-CoA-dehydrogenase deficiency) • Glutaric acidaemia type I • Holocarboxylase synthase deficiency • Isovaleric acidaemia • Methylmalonic acidaemia (cobalamin A and B disorders) • Methylmalonic acidaemia (cobalamin defects C, D v2) • Methylmalonic acidaemia (methylmalonyl-CoA mutase) • Propionic acidaemia |
Other | • Cystic fibrosis • Spinal muscular atrophy • Severe combined immunodeficiency • (Classic) galactosaemia • Other galactosaemias (epimerase, kinase, mutarotase deficiencies) |
Source: Dept. of Health and Aged Care
Newborn bloodspot screening process
The total screening process outlines the steps a family goes through when participating in the program.15 This includes awareness of the program, sample collection, result reporting, and potential referral for further testing or management.
While the screening program itself focuses on identifying conditions and providing initial information, it also aims to support families to access necessary services beyond the program’s scope, such as diagnostic testing and psychological support.16 The total screening process in the NBS program consists of three main phases (see figure 1).
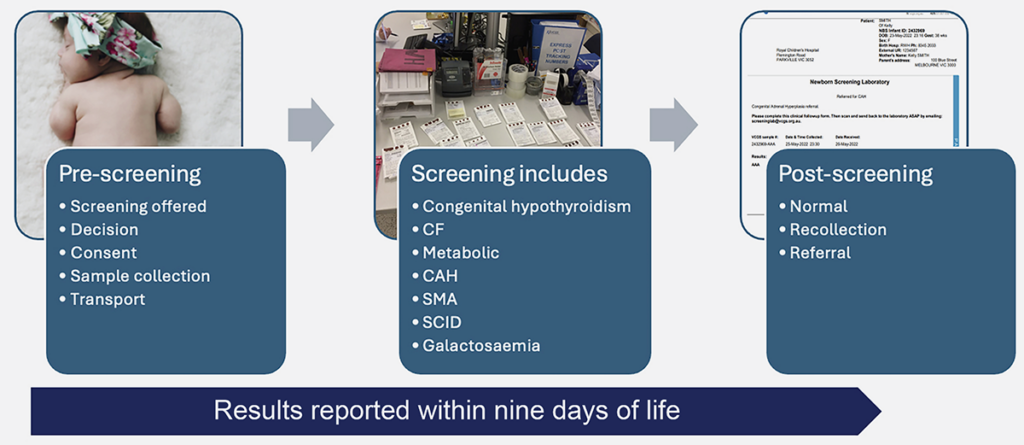
Figure 1. The three phases of the total screening process.
Pre-screening
This involves obtaining informed consent from parents, collecting the blood sample, and transporting the dried blood spot card to the laboratory.17,18 Australian families have the right to consent to or refuse screening on behalf of their baby.
Midwives are asked to provide comprehensive information and opportunities for questions during antenatal visits to ensure informed decision-making.19 Even though families can refuse screening, efforts should be made to facilitate informed consent due to the low-risk nature of screening and potential to prevent serious disability or death.
Proper sample collection and timely transportation are vital for accurate results and timely communication of screening outcomes (see figure 2).18
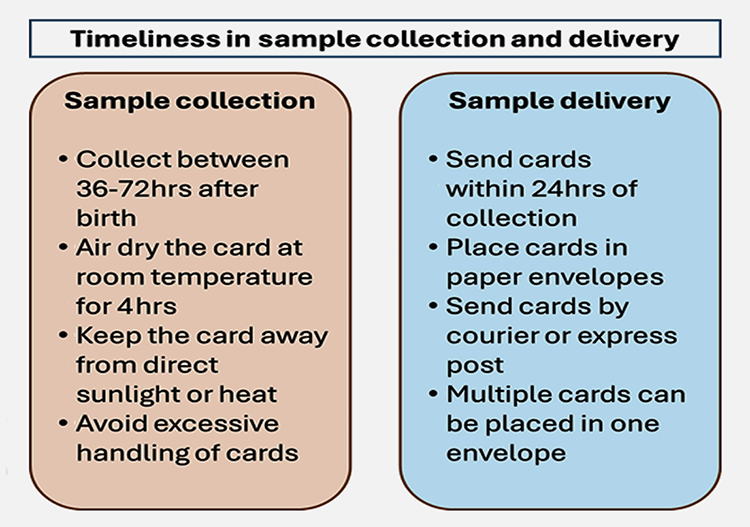
Adapted from Victorian newborn bloodspot screening program information
These parameters are monitored by the maternity service providers, NBS testing lab and the relevant state and territory health departments.
The Department of Health and Aged Care compares the performance of health services to the NBS program’s recommended targets, which involves the number of samples collected within the time frame, timely arrival of the sample to the testing organisation and the quality of the sample collected.13
Screening
The dried blood spots for analysis are sent to designated laboratories that use advanced techniques such as tandem mass spectrometry, which allows for simultaneous screening of multiple conditions.20,21 Initial screening analyses the blood samples for a range of metabolic, endocrine and genetic disorders. This includes testing for specific biomarkers that may indicate the presence of these disorders.
Post-screening
Most babies will screen negative and these results are routinely reported to the relevant maternity service provider. Some babies have mildly abnormal results, and this will lead to a recollection request following the local protocols.
If a baby is screened positive for a potential disorder, the baby will be referred to the relevant specialist clinic for follow-up and further investigation. This will require additional tests to confirm the diagnosis and may include biochemical tests, genetic testing, or other diagnostic procedures.22 If a disorder is confirmed, a care plan is developed, which may include treatment, specialist referrals and ongoing monitoring.
Current challenges
While NBS programs offer significant health benefits, they face challenges in relation to policy, legal frameworks, and the complexities of screening for rare genetic disorders.2,23 One significant issue is the low incidence of many newly proposed disorders for inclusion in the NBS. This complicates the establishment of effective screening protocols.
Additionally, the natural history of some screen-positive cases remains unpredictable, creating unique difficulties in planning NBS services for rare genetic disorders.24 To address these challenges, it is crucial to define the clinical and biochemical phenotypes of suitable target disorders for NBS. This requires appropriate clinical validation of cut-off values, which can only be achieved through multicentre international collaboration to secure sufficient evidence.23,25,26
The integration of new technologies and treatments into NBS programs is another challenge.2 As medical science advances, more sophisticated screening methods are being proposed, including genomic sequencing.27
One major issue is the current cost and turnaround time associated with genomic sequencing.28 The technology and infrastructure required for genomic testing are expensive, and securing sustainable funding is a significant hurdle.
Additionally, extensive training is needed for health professionals to interpret and communicate genomic data effectively. Incorporating these advances requires substantial evidence, funding, and co-ordination among various stakeholders. It is a complex and ongoing task to ensure that all new additions to the screening list are backed by robust clinical validation and that the necessary infrastructure is in place for their implementation.
While genomic sequencing offers great potential, it may add to existing challenges related to consent, data management and the secondary use of samples.29,30 Substantial resources are required to manage informed parental consent, handle large volumes of genomic tests quickly and cost-effectively, and ensure access to multidisciplinary care for asymptomatic newborns.29,31
Effective data storage and access systems, along with rigorous performance monitoring and evaluation, are also essential. Separating genomic NBS from standard NBS may help mitigate risks and support the development of new pathways for education and informed consent.32
Beyond genomics, advances in data integration and health information systems will significantly influence the future of NBS. Enhanced data sharing between hospitals, laboratories, and public health agencies can streamline the screening process and improve outcomes.33
Initiatives like the Newborn Screening Translational Research Network in the US and the European Union Network of Experts on Newborn Screening promote collaboration and data sharing among various stakeholders, including hospitals, laboratories and researchers, to improve the quality and efficiency of NBS programs.34,35 Additionally, the Global Alliance for Genomics and Health is developing data sharing frameworks applicable to NBS programs worldwide, promising exciting advances in the field.36
The role of GPs
GPs play an important role in the NBS program, particularly in the follow-up of abnormal results. As the primary point of contact for many parents, GPs play a key part in communicating NBS results to parents, offering clear explanations and guidance.
If a family has a history of genetic or metabolic disorders, it is important to be aware of the specific conditions that are included in the local NBS program. The state or territory health department can be contacted about an infant’s bloodspot results and for information about additional screening that may be available.
Effective collaboration with midwives, paediatricians and other healthcare providers is essential in creating an effective care pathway for newborns identified with conditions through NBS. Establishing a network with specialists, genetic counsellors and other professionals can help facilitate timely referrals and co-ordinated care.
The Australian experience with spinal muscular atrophy screening, whereby efficient referral pathways and models of care have been developed, highlights the importance of such collaboration. This approach ensures timely access to specialist care, social support and optimising opportunities for early intervention.
Additionally, incorporating education about NBS into prenatal care can help parents feel more informed and prepared for the screening process. It is also important to confirm that an NBS sample has been collected for the child during the postnatal visit to ensure the screening has been completed. This proactive approach enhances the overall effectiveness of the NBS program.
Conclusions
Australia has had successful NBS programs running for almost 60 years. This has saved many lives and reduced the morbidity of disease from early detection.
Until recently, the importance and value of NBS has been underappreciated. Now as we look to the future of NBS, it clearly will represent a significant advance in personalised medicine, utilising novel genetic technologies to transform traditional approaches to diagnosis and management.
This paradigm shift not only benefits individuals but also addresses broader ethical, social, epidemiological and reproductive considerations. The push for earlier and more effective screening methods aligns with international best practice, which advocates for public health measures to support personalised care for rare metabolic diseases.
Timely diagnosis through NBS empowers families with health choices, prepares affected children for emerging therapies, facilitates early rehabilitation and provides essential psychosocial support. For the healthcare provider, keeping abreast of changes in NBS will ensure appropriate guidance is provided to families.
Dr Mercy Thomas (PhD) is a senior lecturer in the school of nursing and health sciences at Swinburne University of Technology, Melbourne, and an honorary research fellow at The Royal Children’s Hospital, Melbourne, and Victorian Clinical Genetics Services, the Murdoch Children’s Research Institute, Melbourne.
Associate Professor Ronda Greaves is the deputy head of biochemical genetics at Victorian Clinical Genetics Services, Murdoch Children’s Research Institute, Melbourne, and an honorary principal fellow in the department of paediatrics at the University of Melbourne.
References on request from Dr Kate Kelso.