Bacteriophage renaissance: Could ‘hungry’ viruses save us from antibiotic resistance?
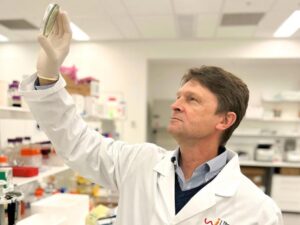
“Long before mammals evolved, bacteria and viruses were fighting it out on this planet.
“Some viruses adapted to live quietly in bacteria.
“But a lot of them eat bacteria every time they meet them, like a horde of hungry teenagers.”
Professor Jon Iredell is referring to bacteriophages, which are viruses that infect bacteria but pose no threat to humans.
The Sydney infectious diseases physician and microbiologist says these “little specialists” can be harnessed to fight hard-to-treat bacterial infections and overcome antimicrobial resistance.
“The trick is for the clinician to match the virus with the bacteria it likes to eat,” he says.
Bacteriophages — phages for short — attack bacteria in the same way that viruses attack human cells.
First, they attach to the bacterial cell wall before injecting it with genetic material to create viral DNA, RNA and proteins, which subsequently form new phages.
Once enough phages have been created, the cell wall bursts open and the viruses are released into the surrounding environment to continue their bacteria hunt.
Phage therapy is already used to treat patients with infections that fail to respond to conventional treatments, but requires clinicians to go through the TGA’s Special Access Scheme.
“That means you cannot really use it unless you can convince the TGA you have done everything that can possibly be done, that the person is still in terrible need and at risk of losing life or limb,” says Professor Iredell, from the University of Sydney.
“On the plus side, it is great to have something to reach for when things look desperate.
“But the downside is that it has not yet been through all those classic clinical trials we would normally expect to have.”
Despite these constraints, Professor Iredell believes phages could succeed where antimicrobials fail.
“For example, if you have an implanted prosthetic device — like a hip joint, a knee joint or a pacemaker — and you get an infection, antibiotics really struggle, but phages offer something extra.”
But phage therapy is not a new concept.
Phages were discovered by British bacteriologist Dr Frederick Twort in 1915, then separately by self-taught French microbiologist Félix d’Hérelle in 1917.
Their therapeutic use dates back to 1919 — a decade before the discovery of penicillin — when Mr d’Hérelle ended an outbreak of avian typhoid in chickens
He cultured phages from one hen that survived the infection and discovered the same phage in the excreta of all other surviving hens on the poultry farm.
He was able to cure infected chickens by adding the phage cultures to their drinking water.
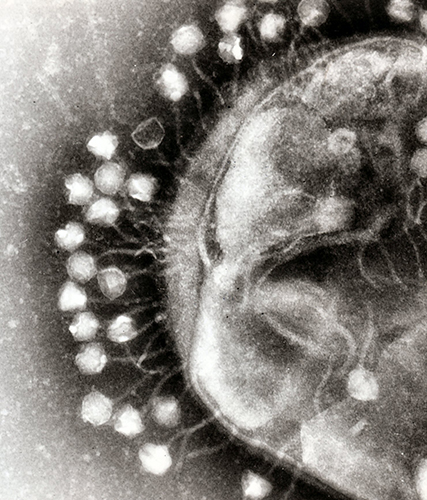
Then in 1927, he used phage therapy in 74 people with Asiatic cholera in India; those treated with phages had an overall mortality rate of 8% compared with 63% for 124 patients treated by other means.
“It was used particularly in France and Eastern Europe, but when the Iron Curtain came down after the Second World War, the factories of the capitalist West started making antibiotics,” Professor Iredell says.
Phage therapy continued to develop in Eastern Europe and India, with “predator” phages matched with “prey” bacteria, but fell out of favour in Western countries because of the wide availability of antibiotics.
Another problem was the bacteriophages’ “fussy” diet.
“People began to realise that phages kept missing the target,” says Professor Iredell.
“Without DNA sequencing tools and fancy laboratory diagnostic testing systems, it was really hard to actually choose the right phage for the right bacteria.
“But now, of course, it is relatively straightforward to do that.”
Against the backdrop of rising antimicrobial resistance, he believes that phage therapy will become mainstream over the next five years.
“We have already got the product available, and we are already using it successfully.
“We have just got to figure out a few things around cost of production, barriers to investment and the regulatory framework.”
This includes working with the TGA and the Office of the Gene Technology Regulator to develop a phage therapy framework “that is fit for purpose”.
One of the key barriers to registration has been the so-called phage therapy paradox.
According to Phage Australia — a network of phage clinicians and scientists, of which Professor Iredell is a member — traditional clinical trials are ill-suited for personalised therapeutics where the product differs based on patient needs.
To get around this, they came up with STAMP — or Standardised Treatment and Monitoring Protocol for Adults and Paediatric Patients — to evaluate the clinical protocol for administering and monitoring phage therapy rather than the phage product itself.
In other words, it means Phage Australia researchers can treat more patients with personalised phages “without sacrificing the standardised dosing and outcome monitoring that come with clinical trials”.
Professor Iredell, who is director of the Westmead Institute of Medical Research (WIMR), says STAMP is being scrutinised as part of an open-label trial with the aim of recruiting 50-100 patients over five years.
“What we are studying is the feasibility of administering phage therapy, testing for safety and for bioavailability and pharmacokinetics. And it has allowed us to get a pretty good idea of what the right dose should be,” he says.
The trial is open to any indication, route of administration or bacteria, but patients must receive TGA approval in advance or be eligible under category A of the Special Access Scheme.
An infectious diseases specialist must confirm there are no other treatment options before the patient is referred to the trial.
“We then test to see if we have got a match with a phage, and if we do, we seek informed consent from the patient and treat it like a normal clinical trial,” Professor Iredell explains.
“We have treated about 30 people here in Australia — almost all of them at Westmead, a couple in Melbourne.
“We have treated people intravenously, we have used a fair bit of nebulised therapy and we have used a little bit of local administration into sites — for example, into a wound or joint.”
These included a seven-year-old girl with chronic multi-drug resistant osteoarticular Pseudomonas aeruginosa infection in her fracture–dislocated ankle.
In 2021, after three months of IV colistin and aztreonam failed to prevent infection following surgical debridement, she received P. aeruginosa IV phage therapy alongside another nine months of antibiotics.
Within seven weeks of the phage therapy, she was able to bear weight without pain for the first time.
Five months on from completing antibiotic treatment, she had no infective symptoms.
In a separate trial involving 13 patients with resistant Staphylococcus aureus bacteraemia and septic shock, eight patients showed clinical improvement within two weeks of IV therapy with three Myoviridae phages.
“Of course, when you introduce a new therapy, you tend to use it when you are absolutely desperate and your back is against the wall,” Professor Iredell says.
“That is when it is most likely to fail.
“But even in the context of runaway antimicrobial-resistant infections or infections that people have failed to treat for months and the patient is now dying, it is working remarkably well.”
In July, the NSW Government announced it would invest $3.5 million over two years to boost phage therapy production via Professor Iredell’s team at the WIMR.
“We know there is a global manufacturing bottleneck due to the very few facilities worldwide that can manufacture high-quality phage products,” NSW Minister for Medical Research David Harris said at the time.
“This is severely limiting supply, which impacts the delivery of this treatment.”
Professor Iredell’s lab contains a collection of more than 300 registered phages, which are tested against bacterial samples from patients to identify the most effective treatment.
If a match is found, the team can manufacture a treatment cocktail by culturing a few suitable phages and combining them in a phosphate-buffered saline solution.
“When it is produced, it looks very much like an antibiotic,” Professor Iredell says.
“It is the same kind of dose as an antibiotic, and we typically use it for 1-2 weeks.”
It is a labour-intensive process, taking days or weeks depending on how easily the phages can be sourced and produced.
The use of multiple phages, rather than just one, plus co-administration with antibiotics, is all designed to reduce the likelihood of the bacteria surviving to develop resistance.
“The way to use phage therapy is to overwhelm the bacteria — this ebb and flow of the balance between predator and prey is normal.”
If the required phage is not in the WIMR’s collection, the team can ask for help from a global network of phage labs with their own bespoke collections.
Broadly speaking, however, phages can be found anywhere that the target bacteria grow.
“Really unusual bugs might need really unusual phages, so they might take more time to track down, while common bugs, like golden staph or pseudomonas, might already have a phage to hand,” Professor Iredell says.
“For example, let’s say you want to kill bacteria that normally live in the human gut: the best place to find the thing that normally eats them is in the water treatment works.
“If you want to find something to kill a bacterium like pseudomonas, that lives in the S-bend of a sink or in the river, the best place to find it is in soils and water.”
Read more: Antimicrobial resistance is far more dangerous than COVID-19, so what can we do?